Drones, or Unmanned Aerial Vehicles (UAVs), represent a remarkable blend of aerodynamics, physics, and advanced engineering. In this discussion, our focus is on quadcopter drones, which highlight these principles in action. Whether you’re a hobbyist, a professional, or simply curious, exploring the physics behind quadcopters can deepen your appreciation for these incredible machines.
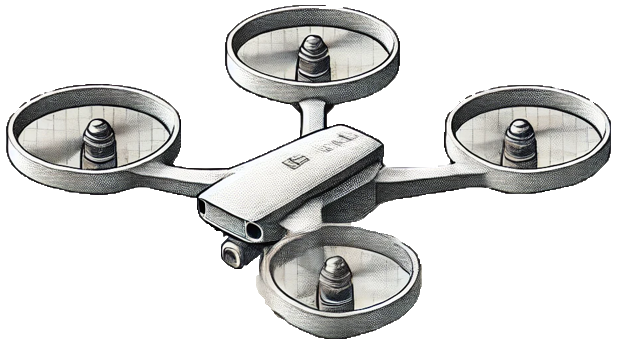
What This Article Covers:
This article explores the fascinating physics behind drones, delving into the forces that keep them airborne and the mechanics of how their propellers generate lift and thrust. It also examines the stability and control mechanisms that enable precise movements and discusses the physics of wake turbulence created by spinning propellers. Together, these topics provide a comprehensive understanding of the principles that govern drone flight.
The Core Principles of Drone Flight
At the heart of a drone’s operation is Newton’s Third Law of Motion: For every action, there is an equal and opposite reaction. Specifically, as drone propellers push air downwards, they create an upward lift force. Consequently, this lift force allows drones to hover, ascend, or descend with remarkable precision. Moreover, by adjusting the speed of the propellers, drones can finely control their movements and maintain stability during flight.
Key Forces and Components of Forces in Drone Flight:
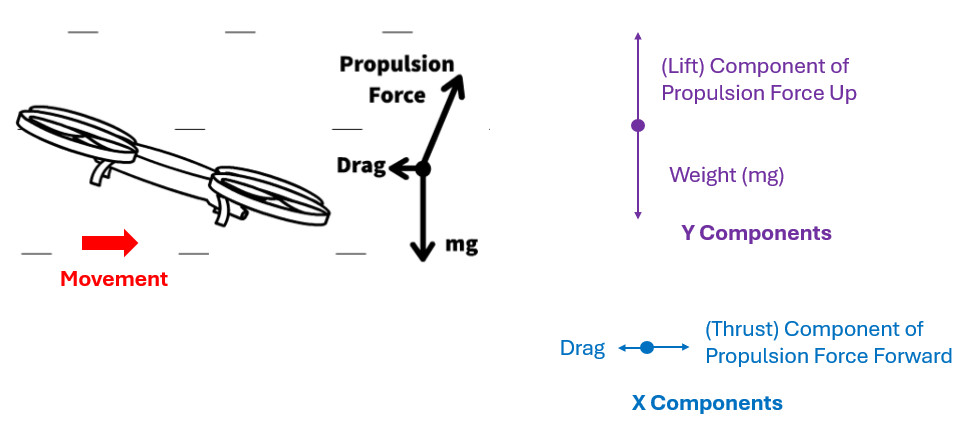
1. Propulsion force: The propellers of a quadcopter are curved rotate in a way that they push air in one direction.
2. Weight: The downward pull of the earth on the drones mass equal to the drones weight (Fg = mg).
3. Drag: Air resistance that opposes motion.
4. Lift: Generated by the propeller propulsion force, lift directed upwards counters gravity to keep the drone airborne.
5. Thrust: The propulsion force of the propellers, when the quadcopter drone is at an angle, can also have a component horizontally we call thrust.
To maintain stable flight, these forces must be perfectly balanced—a feat achieved through the drone’s onboard flight controller and gyroscopic sensors.
Propellers and Lift Generation
Drone propellers function like miniature airplane wings, generating lift by displacing air. The amount of lift depends on the rotational speed and blade angle, which dictate how much air is moved. As a result, larger propellers or faster rotation speeds produce greater lift, enabling drones to carry heavier payloads.
Find more on drone aerodynamics on a video linked here.
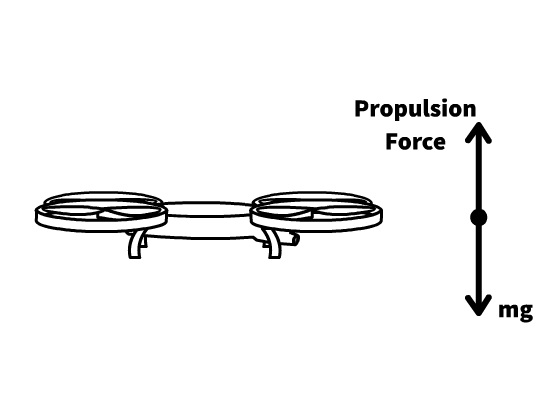
A drone must be in static equilibrium if it is hovering meaning there is no net force on a drone. The propulsion force in this case all lift up would have to be equal in magnitude to the weight (Fg = mg) of the drone down.
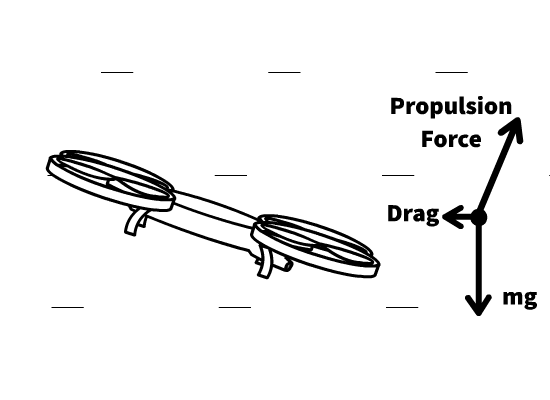
When a drone is moving forward, it could be in dynamic equilibrium if it maintains a constant velocity. In this case, the propulsion force is tilted forward, with its vertical component balancing the weight of the drone (Fg = mg) to maintain altitude, and its horizontal component perfectly counteracting drag, resulting in no net force. Alternatively, if the drone is accelerating forward, it is not in equilibrium because there is a net force in the direction of motion. This net force occurs when the horizontal component of the propulsion force exceeds the opposing drag, causing the drone to accelerate.
Stability and Control Mechanisms
Stability in a drone relies on gyroscopes and accelerometers that detect changes in orientation and motion. To maintain balance, the flight controller adjusts the speed of individual motors. These adjustments ensure the drone remains level and stable during flight.
Yaw, Pitch, and Roll:

Yaw, pitch, and roll are the three primary movements that control a drone’s orientation and direction. A Yaw refers to the rotation around the vertical axis, allowing the drone to turn left or right. Pitch involves tilting the drone forward or backward, enabling forward or backward movement. Roll, on the other hand, tilts the drone sideways, facilitating lateral motion to the left or right. Together, these movements give drones the agility and precision needed for complex maneuvers and stable flight.
How Torque Controls Vertical Rotation in Quadcopters
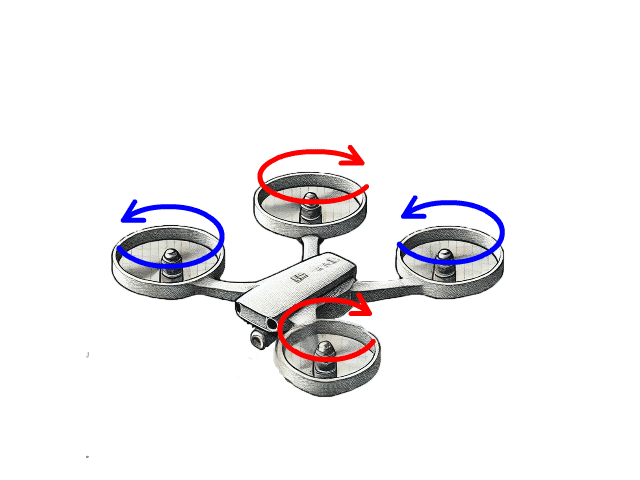
Torque plays a key role in controlling the vertical rotation, or yaw, of a quadcopter drone. Each spinning propeller generates torque as a reaction to the motor’s rotation. To maintain stability and prevent unintended yaw, the propellers must counterbalance each other’s torque. Consequently, adjacent propellers rotate in opposite directions—clockwise and counterclockwise—to achieve this balance. When the drone doesn’t need to rotate, the torques cancel each other out, resulting in a net torque of zero. Therefore, the drone can hover or move straight without spinning.
However, when the drone needs to yaw, it adjusts the speeds of specific pairs of propellers to create a torque imbalance. For instance, speeding up two propellers that spin in one direction while slowing down the other two generates a net torque in the desired direction of rotation. As a result, the drone begins to yaw, or rotate about its vertical axis. By carefully controlling the speeds of its propellers, the drone can achieve precise yaw movements. This allows it to perform smooth turns and maintain its orientation during flight. Ultimately, this torque-based control is essential for the maneuverability and stability of quadcopters.
The Physics of Drones Wake Turbulence
One of the less-discussed aspects of drone flight is wake turbulence, a phenomenon caused by the spiraling airflows generated as drone propellers spin. These airflows can interfere with nearby objects or other drones, particularly in close-proximity operations. Several factors influence the intensity and behavior of this turbulence, including the size and shape of the propellers, their rotational speed, the density of the surrounding air, and environmental conditions. Understanding and managing wake turbulence is crucial for optimizing drone performance and ensuring safe operations.
Wake turbulence becomes especially critical in drone swarms or during close-proximity flights. Engineers use computational fluid dynamics (CFD) simulations to optimize propeller designs and reduce turbulence.
Physics of Drones: Energy Efficiency and Battery Life
Physics plays a crucial role in determining how efficiently drones consume energy. Key factors influencing energy efficiency include the drone’s weight, with heavier drones requiring more lift and, consequently, more energy; propeller efficiency, where optimized blade designs minimize energy loss; and aerodynamics, as streamlined frames help reduce drag. To enhance efficiency, manufacturers commonly utilize lightweight materials such as carbon fiber and design drones with aerodynamic features to minimize drag forces, ensuring optimal performance and prolonged battery life.
Drones in Action: Applications of Physics
The principles of drone physics extend beyond theory to power a wide range of practical applications. In aerial photography, drones rely on stable flight to capture high-quality shots with precision. For delivery services, the balance of lift and thrust enables drones to carry and transport heavy payloads efficiently. In agriculture, drones utilize optimized flight paths to evenly spray crops, enhancing productivity and reducing resource waste. These applications showcase the real-world impact of the physics that govern drone operation.
Physics of Drones Conclusion: The Beauty of Drone Physics
Physics of drones is a perfect blend of natural forces and human ingenuity. Specifically, by mastering the principles of lift, thrust, stability, and wake turbulence, drones can achieve remarkable feats across various fields. Furthermore, this intricate balance of forces highlights the impressive engineering that powers their versatility and precision.